Definición geométrica de andamios metálicos para posibles aplicaciones en ingeniería de tejidos
DOI:
https://doi.org/10.17981/ingecuc.15.1.2019.02Palabras clave:
modelo digital, definición geométrica, andamios metálicos, ingeniería de tejidosResumen
Introducción: El diseño de estructuras porosas tipo andamio en ingeniería de tejidos, se direcciona hacia el desarrollo de elementos que promuevan la consolidación ósea, estabilizando los fragmentos tisulares en dispositivos de fijación biodegradable.
Objetivo: Obtener un modelo tridimensional digital para un metal celular que asemeje la morfología ósea cortical y trabecular, con características como geometría, tamaño de poro, porosidad y recubrimiento tipo piel, además de brindar una base para la materialización de estructuras que mejoren la regeneración ósea y faciliten el control de las propiedades mecánicas del andamio para los defectos biológicos de su aplicación.
Metodología: Se presenta un código paramétrico de modelado 3D, mediante la definición de una geometría regular uniforme con una porosidad y tamaño de poro adecuadas, atendiendo a la esencia de los metales celulares y complementada con un cuerpo de recubrimiento tipo piel que envuelve el modelo tridimensional, para elevar la rigidez y la resistencia mecánica del andamio; además de viabilizar el mecanizado de geometrías propias y permitir aislamiento y protección para los casos en los que se requiera.
Resultados: Se generó el desarrollo de dos modelos digitales para metales celulares con condiciones morfológicas complejas, permitiendo una buena interrelación de parámetros geométricos para la proliferación celular y una respuesta favorable a la solicitación estructural en aplicaciones de ingeniería de tejidos.
Conclusiones: El modelo diseñado evidencia la posibilidad de aplicarse al desarrollo de alternativas de fijación ósea, que disminuyan la respuesta inflamatoria, eviten intervenciones secundarias y reduzcan las tasas de rechazo a los elementos actualmente utilizados para tratar afecciones osteomusculares.
Descargas
Citas
G. Falke, y A. Atala, “Reconstrucción de tejidos y órganos utilizando ingeniería tisular”, Arch Argent Pediatr, vol. 98, no. 2, pp. 103–115, Jan. 2000. Retrieved from: http://evunix.uevora.pt/~fcs/bioh16.pdf
C. García, y D. Ortega, “Elementos de Osteosíntesis de uso Habitual en Fracturas del Esqueleto Apendicular: Evaluación Radiológica", Rev. Chil. Radiol., vol. 11, no. 2, pp. 58–70, Jan. 2005. http://dx.doi.org/10.4067/S0717-93082005000200005
T. Albrektsson and C. Johansson, “Osteoinduction, osteoconduction and osseointegration”, Eur. Spine J., vol. 10, no. 2, pp. 96–101, Oct. 2001. http://dx.doi.org/10.1007/s005860100282
N. Jasmawati, J. Djuansjah, M. Kadir and I. Sukmana, “Porous Magnesium Scaffolds for Bone Implant Applications: A Review”, Advanced Materials Research, vol. 1125, no. 1, pp. 437–440, Oct. 2015. https://doi.org/10.4028/www.scientific.net/AMR.1125.437
M-Q. Cheng, T. Wahafu, G-F. Jiang, W. Liu, Y-Q. Qiao, X-C. Peng, T. Chen, X-L. Zhang, G. He & X-Y. Liu, “A novel open-porous magnesium scaffold with controllable microstructures and properties for bone regeneration”, Sci. Rep., vol. 6, no. 1, p. 24134, Apr. 2016. https://doi.org/10.1038/srep24134
Z. Zhen, T-F. Xi, and Y-F. Zheng, “A review on in vitro corrosion performance test of biodegradable metallic materials”, Trans. Nonferrous Met. Soc. China, vol. 23, no. 8, pp. 2283–2293, Aug. 2013. https://doi.org/10.1016/S1003-6326(13)62730-2
J. Mitra, G. Tripathi, A. Sharma and B. Basu, “Scaffolds for bone tissue engineering: role of surface patterning on osteoblast response”, RSC Adv., vol. 3, no. 28, pp. 11073–11094, 2013. https://doi.org/10.1039/C3RA23315D
K-J. Kim, S. Choi, Y. Sang Cho, S-J. Yang, Y-S. Cho and K. K. Kim, “Magnesium ions enhance infiltration of osteoblasts in scaffolds via increasing cell motility”, J. Mater. Sci. Mater. Med., vol. 28, no. 6, pp. 1–7, Jun. 2017. https://doi.org/10.1007/s10856-017-5908-5
A. R. Boccaccini, U. Kneser and A. Arkudas, “Scaffolds for vascularized bone regeneration: advances and challenges”, Expert Rev. Med. Devices, vol. 9, no. 5, pp. 457–460, Sept. 2012. https://doi.org/10.1586/erd.12.49
V. Karageorgiou and D. Kaplan, “Porosity of 3D biomaterial scaffolds and osteogenesis”, Biomaterials, vol. 26, no. 27, pp. 5474–5491, Sept. 2005. https://doi.org/10.1016/j.biomaterials.2005.02.002
L. D. Albrecht, S. W. Sawyer, and P. Soman, “Developing 3D Scaffolds in the Field of Tissue Engineering to Treat Complex Bone Defects,” 3D Print. Addit. Manuf., vol. 3, no. 2, pp. 106–112, Jun. 2016. https://doi.org/10.1089/3dp.2016.0006
K. Alvarez and H. Nakajima, “Metallic Scaffolds for Bone Regeneration”, Materials, vol. 2, no. 3, pp. 790–832, Jul. 2009. https://doi.org/10.3390/ma2030790
M. Tarik, I. Gibson and X. Li, “State of the art and future direction of additive manufactured scaffolds-based bone tissue engineering”, Rapid Prototyp. J., vol. 20, no. 1, pp. 13–26, Jan. 2014. https://doi.org/10.1108/RPJ-03-2012-0023
J. R. Caeiro, P. González and D. Guede, “Biomecánica y hueso (y II): Ensayos en los distintos niveles jerárquicos del hueso y técnicas alternativas para la determinación de la resistencia ósea”, Rev. Osteoporosis Metab. Min., vol. 5, no. 2, pp. 99–108, Jun. 2013. http://dx.doi.org/10.4321/S1889-836X2013000200007
P. J. Prendergast and P. E. McHugh, “Topics in bio-mechanical engineering”, in 1st Symposium on Biomechanical Engineering, Dublin, Ireland, Nov. 27, 2004.
X-Y. Zhang, G. Fang, and J. Zhou, “Additively Manufactured Scaffolds for Bone Tissue Engineering and the Prediction of their Mechanical behavior: A review”, Materials, vol. 10, no. 1, pp. 1–28, Jan. 2017. https://doi.org/10.3390/ma10010050
G. Maliaris, “Mechanical and fracture behaviour of cellular materials with regular and random lattice structures under various compressive velocities”, in 4th International Conference of Engineering Against Failure (ICEAF IV), Skiathos, Greece, Jun. 24–26, 2015.
J. Banhart, “Manufacture, characterisation and application of cellular metals and metal foams”, Prog. Mater. Sci., vol. 46, no. 6, pp. 559–632, Dec. 2001. https://doi.org/10.1016/S0079-6425(00)00002-5
P. Pinto, N. Peixinho, D. Soares and F. Silva, “Process development for manufacturing of cellular structures with controlled geometry and properties,” Mater. Res., vol. 18, no. 2, pp. 274–282, Apr. 2015. https://doi.org/10.1590/1516-1439.286614
Č. Jaroslav and D. Vojtěch, “Characterization of porous magnesium prepared by powder metallurgy - influence of powder shape”, Manuf. Technol., vol. 14, no. 3, pp. 271–275, Jan. 2014.
M. Velasco y D. Garzón, “Implantes Scaffolds para regeneración ósea. Materiales, técnicas y modelado mediante sistemas de reacción-difusión”, Rev. Cubana de Invest. Biomed., Vol. 29, no.1, pp. 140–154, Mar. 2010. Recuperado de http://www.bvs.sld.cu/revistas/ibi/vol_29_1_10/ibi08110.htm
F. Djamaluddin, S. Abdullah, A. Ariffin, and Z. Nopiah, “Finite element analysis and crashworthiness optimization of foam-filled double circular under oblique loading”, Lat. Am. J. Solids Struct., vol. 13, no. 11, pp. 2176–2189, Nov. 2016. https://doi.org/10.1590/1679-78252844
A. Uzun, H. Karakoc, U. Gokmen, H. Cinici, and M. Turker, “Investigation of mechanical properties of tubular aluminum foams”, Int. J. Mater. Res., vol. 107, no. 11, pp. 996–1004, Nov. 2016. https://doi.org/10.3139/146.111430
N. Chantarapanich, P. Puttawibul, S. Sucharitpwatskul, P. Jeamwatthanachai, S. Inglam, and K. Sitthiseripratip, “Scaffold Library for Tissue Engineering: A Geometric Evaluation”, Comput. Mat. Methods Med., vol. 2012, no. 1, pp. 1–14. Sept. 2012. http://dx.doi.org/10.1155/2012/407805
F. Zwarts, "Size effects in cellular solids", Ph.D. dissertation, Dept. Mat. Nat. Sci. RUG, Groningen, 2007. Retrieved from: https://www.uni-due.de/~hm0014/Cosserat_files/tekoglu_thesis06.pdf
H. Kanahashi, T. Mukai, T. G. Nieh, T. Aizawa, and K. Higashi, “Effect of Cell Size on the Dynamic Compressive Properties of Open-Celled Aluminum Foams”, Mater. Trans., vol. 43, no. 10, pp. 2548–2553, Sept. 2002. https://doi.org/10.2320/matertrans.43.2548
R. Oftadeh, M. Perez-viloria, J. Villa-camacho, A. Vaziri, and A. Nazarian, “Biomechanics and Mechanobiology of Trabecular Bone: A Review”, J. Biomech. Eng., vol. 137, no. 1, pp. 1–15, Dec. 2015. https://doi.org/10.1115/1.4029176
C. Misch, Z. Qu and M. Bidez, “Mechanical properties of trabecular bone in the human mandible: implications for dental implant treatment planning and surgical placement”, J. Oral Maxillofac. Surg., vol. 57, no. 6, pp. 700–706, Jun. 1999. https://doi.org/10.1016/S0278-2391(99)90437-8
F. Warmuth, F. Osmanlic, L. Adler, M. A. Lodes, and C. Körner, “Fabrication and characterisation of a fully auxetic 3D lattice structure via selective electron beam melting”, Smart Mater. Struct., vol. 26, no. 2, p. 025013, Dec. 2017. https://doi.org/10.1088/1361-665X/26/2/025013
Y. Ding, J. Lin, C. Wen, D. Zhang, and Y. Li, “Mechanical properties, in vitro corrosion and biocompatibility of newly developed biodegradable Mg-Zr-Sr-Ho alloys for biomedical applications” Sci. Rep., vol. 6, no. 1, pp. 1–10, Aug. 2016. https://doi.org/10.1038/srep31990
S. Ribeiro-Ayeh, “Finite element modelling of the mechanics of solid foam materials”, PhD dissertation, KTH, Dept. Aer. Veh. Eng., Stockholm, 2005. Retrieved from: http://kth.diva-portal.org/smash/get/diva2:7450/FULLTEXT01.pdf
W. S. Thomson, “On the division of space with minimum partitional area”, Acta Math., vol. 11, no. 1, pp. 121–134, Mar. 1887. https://projecteuclid.org/euclid.acta/1485881153
P. J. Veale, “Investigation of the Behavior of Open Cell Aluminum Foam”, M. S. thesis, Dept. Civil. Env. Eng., Mass. Univ., Amherst, 2010. Retrieved from: https://scholarworks.umass.edu/cgi/viewcontent.cgi?article=1527&context=theses
D. M. Robertson, L. Pierre and R. Chahal, “Preliminary Observations of Bone Ingrowth into Porous Materials”, J Biomed Mater Res., vol. 10, no. 3, pp. 335–344, May. 1976. https://doi.org/10.1002/jbm.820100304
V. Guneta, J. K. Wang, S. Maleksaeedi, Z. M. He, M. T. C. Wong, and C. Choong, “Three-Dimensional Printing of Titanium for Bone Tissue Engineering Applications: A Preliminary Study”, J. Biomimetics, Biomater. Biomed. Eng., vol. 21, no. 1, pp. 101–115, Aug, 2014. https://doi.org/10.4028/www.scientific.net/JBBBE.21.101
F. Bobbert, K. Lietaert, A. Eftekhari, B. Pouran, S. Ahmadi, H. Weinans, and A. Zadpoor, “Additively manufactured metallic porous biomaterials based on minimal surfaces: A unique combination of topological, mechanical, and mass transport properties”, Acta Biomaterialia, vol. 53, no. 1, pp. 572–584, Apr. 2017. https://doi.org/10.1016/j.actbio.2017.02.024.
S. J. Hollister, “Porous scaffold design for tissue engineering”, Nat. Mater., vol. 4, no. 7, pp. 518–24, Jul. 2005. https://doi.org/10.1038/nmat1421
G. Jia, Y. Hou, C. Chen, J. Niu, H. Zhang, and H. Huang, “Precise fabrication of open porous Mg scaffolds using NaCl templates: Relationship between space holder particles, pore characteristics and mechanical behavior”, Mater. Des., vol. 140, pp. 106–113, Feb. 2018. https://doi.org/10.1016/j.matdes.2017.11.064
J. Osorio-Hernández, M. Suarez, R. Goodall, G. Lara-Rodriguez, I. Alfonso, and I. Figueroa, “Manufacturing of open-cell Mg foams by replication process and mechanical properties”, Mater. Des., vol. 64, pp. 136–141, Dec. 2014. https://doi.org/10.1016/j.matdes.2014.07.015
Z. Li and F. Lu, “Bending resistance and energy-absorbing effectiveness of empty and foam-filled thin-walled tubes”, J. Reinf. Plast. Compos., vol. 34, no. 9, pp. 761–768, Apr. 2015. https://doi.org/10.1177/0731684415580329
Robert McNeel & Associates, “Rhinoceros 3D”, noviembre, 2012, [En línea . Disponible en: https://www.rhino3d.com/la/
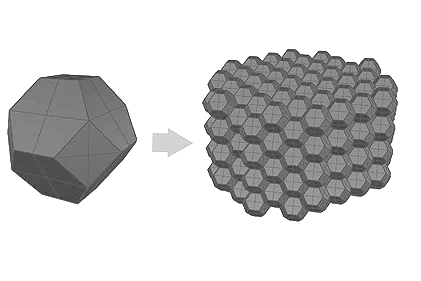
Publicado
Cómo citar
Número
Sección
Licencia
Derechos de autor 2019 INGE CUC

Esta obra está bajo una licencia internacional Creative Commons Atribución-NoComercial-SinDerivadas 4.0.
Los artículos publicados son de exclusiva responsabilidad de sus autores y no reflejan necesariamente las opiniones del comité editorial.
La Revista INGE CUC respeta los derechos morales de sus autores, los cuales ceden al comité editorial los derechos patrimoniales del material publicado. A su vez, los autores informan que el presente trabajo es inédito y no ha sido publicado anteriormente.
Todos los artículos están bajo una Licencia Creative Commons Atribución-NoComercial-SinDerivadas 4.0 Internacional.