CO2 and cost optimization of reinforced concrete footings over a lime-treated soil using modified simulated annealing algorithm
DOI:
https://doi.org/10.17981/ingecuc.16.1.2020.07Keywords:
multi-objective optimization, modified simulated annealing algorithm, spread footing, lime-soilAbstract
Introduction: The design of spread footings over a lime-treated soil is studied as an important topic in geotechnical and environmental engineering. With the emergence and use of algorithms, it is possible to solve optimization problems in engineering, leading, for example, to decreased amounts of materials, time, energy, and work.
Objective: This research aims to optimize the CO2 emission and cost of building spread footings over a treated soil with hydrated lime using the modified simulated annealing algorithm (MSAA).
Method: The parameters for shear strength (cohesion and friction angle) was calculated of a silty soil of the Guabirotuba geological formation of Curitiba (Brazil) stabilized with different lime contents (3, 5, 7 and 9%) at different curing times (30, 90, and 180 days). Then with these parameters, the geometry of the spread footings was optimized with MSAA minimizing the cost and CO2 emissions of their construction. For the design constraint of the structures the ultimate bearing capacity of the soil was used as criteria, the settlements produced by the service load, and the base safety factor
Results: The results show that most of the problems converge to the same solution for costs and CO2 emissions without depending on curing time and lime content used, due to the solutions being restricted primarily by the maximum permissible settlements.
Conclusions: With the increase in lime content, the cohesion of the mixtures increased for all curing times studied ant the friction angle had no major variations in relation to the amount of lime administered or to the curing time employed. Costs and carbon dioxide emissions for spread footing construction converge to the same results. In this sense, 9% lime can be avoided, and small percentages of lime (i.e. 3-5%) are appropriated to ground improvement and reduce the costs of this procedure. On the other hand, the MSAA can be designated as a robust algorithm due to having achieved almost equal results and, in some cases, better results compared with other algorithms to solve problems reported in the literature.
Downloads
References
B. Celauro, A. Bevilacqua, D. L. Bosco and C. Celauro, “Design Procedures for Soil-Lime Stabilization for Road and Railway Embankments. Part 1-Review of Design Methods,” Procedia - Soc. Behav. Sci., vol. 53, no. 3, pp. 754–763, Oct. 2012. https://doi.org/10.1016/j.sbspro.2012.09.925
P. T. Sherwood, Soil stabilization with cement and lime, Crowthorne, UK: TRL, 1993.
N. C. Consoli, M. Bellaver and L. Festugato, “Key parameter for tensile and compressive strength of fibre-reinforced soil–lime mixtures,” Geosynth. Int., vol. 19, no. 5, pp. 409–414, Oct. 2012. https://doi.org/10.1680/gein.12.00026
J. A. Baldovino, E. B. Moreira, W. Teixeira, R. L. S. Izzo and J. L. Rose, “Effects of lime addition on geotechnical properties of sedimentary soil in Curitiba, Brazil,” J. Rock Mech. Geotech. Eng., vol. 1, no. 1, pp. 1–7, Feb. 2017. https://doi.org/10.1016/j.jrmge.2017.10.001
A. A. Al-Rawas, A. W. Hago and H. Al-Sarmi, “Effect of lime, cement and Sarooj (artificial pozzolan) on the swelling potential of an expansive soil from Oman,” Build. Environ., vol. 40, no. 5, pp. 681–687, May. 2005. https://doi.org/10.1016/j.buildenv.2004.08.028
B. Das, Principios de ingeniería de cimentaciones, México, MX: Thompson Editores, 2006.
Y. Wang and F. H. Kulhawy, “Economic Design Optimization of Foundations,” J. Geotech. Geoenvironmental Eng., vol. 134, no. 8, pp. 1097–1105, Aug. 2008. https://doi.org/10.1061/(ASCE)1090-0241(2008)134:8(1097).
M. Khajehzadeh, M. R. Taha, A. El-Shafie and M. Eslami, “Modified particle swarm optimization for optimum design of spread footing and retaining wall,” J. Zhejiang Univ. A., vol. 12, no. 6, pp. 415–427, Jun. 2011. https://doi.org/10.1631/jzus.A1000252
C. V. Camp and A. Assadollahi, “CO2 and cost optimization of reinforced concrete footings using a hybrid big bang-big crunch algorithm,” Struct. Multidiscip. Optim., vol. 48, no. 2, pp. 411–426, Feb. 2013. https://doi.org/10.1007/s00158-013-0897-6
C. V. Camp and A. Assadollahi, “CO2 and cost optimization of reinforced concrete footings subjected to uniaxial uplift,” J. Build. Eng., vol. 3, pp. 171–183, Sep. 2015. https://doi.org/10.1016/j.jobe.2015.07.008
B. Ahmadi-Nedushan and H. Varaee, “Optimal Design of Reinforced Concrete Retaining Walls using a Swarm Intelligence Technique,” present at the 1st Int. Conf. Soft Comput. Technol. Civil, Struct. Environ. Eng., CCP: 92, Stirlingsh., UK, 2009, Paper 26. https://doi.org/10.4203/ccp.92.26
M. Khajehzadeh, M. R. Taha, A. El-Shafie and M. Eslami, “Economic design of retaining wall using particle swarm optimization with passive congregation,” Aust. J. Basic Appl. Sci., vol. 4, no. 11, pp. 5500–5507, Nov. 2010.
M. Khajehzadeh and M. Eslami, “Gravitational search algorithm for optimization of retaining structures,” Indian J. Sci. Technol., vol. 5, no. 1, pp. 1821–1827, Jan. 2012.
V. Yepes, J. Alcala, C. Perea and F. González-Vidosa, “A parametric study of optimum earth-retaining walls by simulated annealing,” Eng. Struct., vol. 30, no. 3, pp. 821–830, Mar. 2008. https://doi.org/10.1016/j.engstruct.2007.05.023
A. Kaveh and A. S. M. Abadi, “Harmony search based algorithms for the optimum cost design of reinforced concrete cantilever retaining walls,” Int. J. Civ. Eng., vol. 9, no. 1, pp. 1–8, Mar. 2011.
A. Kaveh and A. F. Behnam, “Charged System Search Algorithm for the Optimum Cost Design of Reinforced Concrete Cantilever Retaining Walls,” Arab. J. Sci. Eng., vol. 38, no. 3, pp. 563–570, Mar. 2012. https://doi.org/10.1007/s13369-012-0332-0
R. Sheikholeslami, B. G. Khalili and S. M. Zahrai, “Optimum Cost Design of Reinforced Concrete Retaining Walls Using Hybrid Firefly Algorithm,” Int. J. Eng. Technol., vol. 6, no. 6, pp. 465–470, Jan. 2014. https://doi.org/10.7763/IJET.2014.V6.742
C. V. Camp and A. Akin, “Design of Retaining Walls Using Big Bang–Big Crunch Optimization,” J. Struct. Eng., vol. 138, no. 3, pp. 438–448, Mar. 2012. https://doi.org/10.1061/(ASCE)ST.1943-541X.0000461
A. H. Gandomi, A. R. Kashani, D. A. Roke and M. Mousavi, “Optimization of retaining wall design using recent swarm intelligence techniques,” Eng. Struct., vol. 103, pp. 72–84, Jan. 2014. https://doi.org/10.1016/j.engstruct.2015.08.034
A. H. Gandomi, A. R. Kashani, D. A. Roke and M. Mousavi, “Optimization of retaining wall design using evolutionary algorithms,” Struct. Multidiscip. Optim., vol. 55, pp. 809–825, Jul. 2016. https://doi.org/10.1007/s00158-016-1521-3
M. G. Sahab, V. V. Toropov and A. H. Gandomi, “A Review on Traditional and Modern Structural Optimization: Problems and Techniques,” in Metaheuristic Applications in Structures and Infrastructures, 2013, pp. 25–47. https://doi.org/10.1016/B978-0-12-398364-0.00002-4
S. Pezeshk and C. Camp, “State of the art on the use of genetic algorithms in design of steel structures,” Recent Adv. Optim. Struct. Des. pp. 1–31, 2002.
S. K. Das and P. K. Basudhar, “Comparison study of parameter estimation techniques for rock failure criterion models,” Can. Geotech. J., vol. 43, no. 7, pp. 764–771, Jan. 2011. https://doi.org/10.1139/t06-041
C. M. Páramo and O. B. Carrillo, “Solución de problemas de optimización topológica empleando el Algoritmo Simulated Annealing Modificado,” RIMNI, vol. 32, no. 2, pp. 65–69, May. 2016. https://doi.org/10.1016/j.rimni.2014.11.005
C. Millán, O. B. Carrillo and E. M. Romero, “Propuesta y validación de un algoritmo Simulated annealing modificado para la solución de problemas de optimización,” RIMNI, vol. 30, no. 4, pp. 264–270, Dec. 2014. https://doi.org/10.1016/j.rimni.2013.10.003
C. M. Páramo and E. Millán, “Algoritmo simulated annealing modificado para minimizar peso en cerchas planas con variables discretas,” INGE CUC, vol. 12, no. 2, pp. 9–16, Ago. 2016. https://doi.org/10.17981/ingecuc.12.2.2016.01
C. Millan-Paramo, “Modified Simulated Annealing Algorithm for Discrete Sizing Optimization of Truss Structure,” JJCE, vol. 12, no. 4, pp. 683–697, Jan. 2018.
Standard Practice for Classification of Soils for Engineering Purposes (Unified Soil Classification System), ASTM D2487, Am. Soc. Test. Mater, West Conshohocken, USA, 2011. https://doi.org/10.1520/D1238-13
Stardard Test Methods for Liquid Limit, Plastic Limit and Plasticity Index of Soils, ASTM D4318-10, Am. Soc. Test. Mater, West Conshohocken, USA, 2010.
Standard Test Methods for Specific Gravity of Soil Solids by Water Pycnometer 1, ASTM D 854-14, Am. Soc. Test. Mater, West Conshohocken, USA,, 2014.
J. Arrieta, E. Moreira, R. Izzo and J. Rose, “Empirical Relationships with Unconfined Compressive Strength and Split Tensile Strength for the Long Term of a Lime-Treated Silty Soil,” J. Mater. Civ. Eng., vol. 8, no. 30, pp. 06018008, May. 2018. https://doi.org/10.1061/(ASCE)MT.1943-5533.0002378
Cal-hidratada-para-argamassas, ABNT, NBR-7175, Assossiação Bras de Normas Técnicas, GRU, BR, 2003.
Solo - Ensaio de Compactação, ABNT, NBR 7182, Assossiação Bras de Normas Técnicas, GRU, BR, 2016.
Standard Test Method for Direct Shear Test of Soils Under Consolidated Drained Conditions, ASTM D 3080-98, Am. Soc. Test. Mater, West Conshohocken, USA, 2003. https://doi.org/10.1520/D3080-98
G. G. Meyerhof, “Some Recent Research on the Bearing Capacity of Foundations,” Can. Geotech. J., vol. 1, no. 1, pp. 16–26, Jan. 2011, https://doi.org/10.1139/t63-003
J. B. Hansen, “A Revised and Extended Formula for Bearing Capacity,” Geotechnical Institute, Kgs, DK, Bull No 28, 1970.
H. G. Poulos and E. H. Davis, “Elastic solutions for soil and rock mechanics,” Int J Rock Mech Min Sci Geomech Abstr, vol. 11, no. 8, pp. A159, Aug. 1974. https://doi.org/10.1016/0148-9062(74)91768-9
R. Whitman and F. Richart, “Design procedures for dynamically loaded foundations,” J. Soil Mech. Found. Div., vol. 93, no. 6, pp. 169–193, Feb. 1967.
S. Kirkpatrick, C. D. Gelatt and M. P. Vecchi, “Optimization by Simulated Annealing”, Science, vol. 220, no. 4598, pp. 671–680, May. 1983. https://doi.org/10.1126/science.220.4598.671
L. Lamberti, “An efficient simulated annealing algorithm for design optimization of truss structures,” Comput. Struct., vol. 86, no. 19-20, pp. 1936–1953, Oct. 2008. https://doi.org/10.1016/j.compstruc.2008.02.004
A. Vesic, Bearing Capacity for Shallow Foundations, N.Y., USA: Van Nostrand Reinhold Company, Inc., 1975.
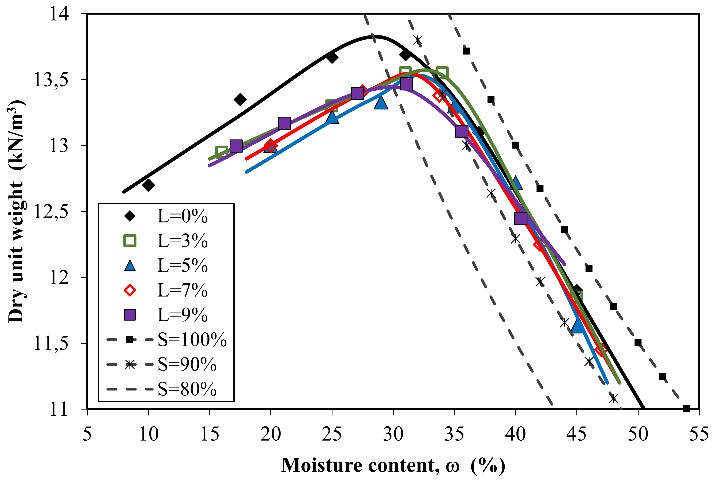
Published
How to Cite
Issue
Section
License
Copyright (c) 2020 INGE CUC

This work is licensed under a Creative Commons Attribution-NonCommercial-NoDerivatives 4.0 International License.
Published papers are the exclusive responsibility of their authors and do not necessary reflect the opinions of the editorial committee.
INGE CUC Journal respects the moral rights of its authors, whom must cede the editorial committee the patrimonial rights of the published material. In turn, the authors inform that the current work is unpublished and has not been previously published.
All articles are licensed under a Creative Commons Attribution-NonCommercial-NoDerivatives 4.0 International License.