Propiedades fisicomecánicas, desempeño y durabilidad de morteros geopoliméricos a base de puzolana natural tipo piedra pómez
DOI:
https://doi.org/10.17981/ingecuc.17.1.2021.17Palabras clave:
piedra pómez, puzolana natural, cemento, morteros, geopolímerosResumen
Introducción: En los últimos años, los investigadores han estado evaluando el uso potencial de una amplia gama de materias primas en la producción de geopolímeros. Este trabajo reporta la conveniencia de utilizar una puzolana natural tipo piedra pómez de Paipa (Colombia) como material de sustitución del cemento (100% de puzolana) en la producción de morteros geopoliméricos.
Objetivo: El objetivo de esta investigación se enfoca en el uso de la piedra pómez como materia prima en la producción de cementos geopoliméricos con el fin de establecer su comportamiento mecánico y durabilidad.
Metodología: Se utilizaron cemento Portland tipo I, puzolana natural y arena como materiales de partida en la preparación de morteros geopoliméricos a partir de la activación alcalina de puzolana natural. Los morteros geopoliméricos fueron preparados y ensayados según las normas del Instituto Colombiano de Normas Técnicas e Instituto Nacional de Vías.
Resultados: Los ensayos en geopolímeros ofrecen resultados alentadores y muestran un buen potencial en la preparación de cemento con puzolana exhibiendo una adecuada durabilidad y resistencia a la compresión relativamente alta a los 28 días para ser aceptado en el desarrollo de nuevos materiales cementantes.
Conclusiones: El uso de puzolana natural en la producción de geopolímeros se vuelve importante por su papel en la durabilidad del mortero en condiciones ambientales extremas y agresivas.
Descargas
Citas
R. Mejía, S. Delvasto & R. Talero, “Permeability properties of cement mortars blended with Silica Fume, Fly Ash, and Blast Furnace Slag,” Corrosion in Tropical Environments, ASTM STP 1399, pp. 190–196, Jan. 2000. https://doi.org/10.1520/STP13562
J. S. Coutinho, “The combined benefits of CPF and RHA in improving the durability of concrete structures,” Cem Concr Compos, vol. 25, no. 1, pp. 51–59, Jan. 2003. https://doi.org/10.1016/S0958-9465(01)00055-5
E. Gartner, “Industrially interesting Approaches to low CO2 Cements,” Cem Concr Res, vol. 34, no. 9, pp. 1489–1498, Sep. 2004. https://doi.org/10.1016/j.cemconres.2004.01.021
F. Puertas, A. Barra, M. F. Gazulla, M. P. Gomez, M. Palacios & S. Martínez-Ramírez, “Ceramic wastes as raw materials in Portland cement clinker fabrication: characterization and alkaline activation,” Mater Const, vol. 56, no. 281, pp. 73–84, 2006. https://doi.org/10.3989/mc.2006.v56.i281.94
M. C. G. Juenger, F. Winnefeld, J.L. Provis & J. H. Ideker, “Advances in alternative cementitious binders,” Cem Concr Res, vol. 41, no. 12, pp. 1232–1243, Dec. 2011. https://doi.org/10.1016/j.cemconres.2010.11.012
A. B. Kizilkanat, D. Oktay, N. Kabay & M. M. Tufekci, “Comparative Experimental Study of Mortars Incorporating Pumice Powder or Fly Ash,” J Mater Civ Eng, vol. 28, no. 2, pp. 1–7, Feb. 2016. https://doi.org/10.1061/(ASCE)MT.1943-5533.0001407
L. Kaizhi, R. Yu, Z. Shui, X. Li, S. Wu, C. Wuo, B. Yu & S. Wu, “Optimization of autogenous shrinkage and microstructure for Ultra-High Performance Concrete (UHPC) based on appropriate application of porous pumice,” Constr Build Mater, vol. 214, pp. 369–381, Jul. 2019. https://doi.org/10.1016/j.conbuildmat.2019.04.089
A.M. Zeyad, A.H. Khan & B.A. Tayeh, “Durability and strength characteristics of high-strength concrete incorporated with volcanic pumice powder and polypropylene fibers,” J Mater Res Technol, vol. 9, no. 1, pp. 806–818, 2020. https://doi.org/10.1016/j.jmrt.2019.11.021
J. Sanjayan and B. Sioulas, “Strength of slag-cement cured in place and in other conditions,” ACI Mater J, vol. 97, no. 5, pp. 603–611, Sep. 2000. Available: https://www.worldcat.org/title/aci-materials-journal/oclc/13846872
T. W. Cheng & J.P. Chiu, “Fire-resistant geopolymer produced by granulated blast furnace slag,” Miner Eng, vol. 16, no. 3, pp. 205–210, Mar. 2003. hhttps://doi.org/10.1016/S0892-6875(03)00008-6
A. Karthik, K. Sudalaimani, C. T. Vijayakumar & S. S. Saravanakumar, “Effect of bio-additives on physico-chemical properties of fly ash-ground granulated blast furnace slag based self-cured geopolymer mortars,” J Hazard Mater, vol. 361, pp. 56–63, Jan. 2019. https://doi.org/10.1016/j.jhazmat.2018.08.078
J.C. Swanepoel & C.A. Strydom, “Utilization of fly ash in a geopolymeric material,” Appl Geochem, vol. 17, no. 8, pp. 1143–1148, Aug. 2002. https://doi.org/10.1016/S0883-2927(02)00005-7
T. Bakharev, “Geopolymeric materials prepared using Class F fly ash and elevated temperature curing,” Cem Concr Res, vol. 35, no. 6, pp. 1224–1232, Jun. 2005. https://doi.org/10.1016/j.cemconres.2004.06.031
A. Fernández-Jimenez & A. Palomo, “Composition and microstructure of alkali activated fly ash binder: Effect of the activator,” Cem Concr Res, vol. 35, no. 10, pp. 1984–1992, Oct. 2005. https://doi.org/10.1016/j.cemconres.2005.03.003
G. Kürklü & G. Görhan, “Investigation of usability of quarry dust waste in fly ash-based geopolymer adhesive mortar production,” Constr Build Mater, vol. 217, pp. 498–506, Aug. 2019. https://doi.org/10.1016/j.conbuildmat.2019.05.104
A. Wongsa, R. Kunthawatwong, S. Naenudon, V. Sata & P. Chindaprasirt, “Natural fiber reinforced high calcium fly ash geopolymer mortar,” Constr Build Mater, vol. 241, Apr. 2020. https://doi.org/10.1016/j.conbuildmat.2020.118143
P.H. Shih & S. Yokohama, “Behaviour of chloride ions in hardened ecocement: New type of Portland cement made from municipal waste incinerator ash,” 11 International Congress on the Chemistry of Cement, ICCC, Durban, SA, pp. 1271–1281, 11-16 May, 2003.
F. Puertas, M.T. Blanco-Varela & T. Vazquez, “Behaviour of cement mortars containing an industrial waste from aluminum refining. Stability in Ca(OH)2 solutions,” Cem Concr Res, vol. 29, no. 10, pp. 1673–1680, Oct. 1999. https://doi.org/10.1016/S0008-8846(99)00157-X
C. Leiva, Y. Luna-Galiano, C. Arenas, B. Alonso-Fariñas & C. Fernández-Pereira, “A porous geopolymer based on aluminum-waste with acoustic properties,” Waste Manag, vol. 95, pp. 504–512, Jul. 2019. https://doi.org/10.1016/j.wasman.2019.06.042
A. Hauser, U. Eggenberger & T. Mumenthaler, “Fly ash from cellulose industry as secondary raw material in autoclaved aerated concrete,” Cem Concr Res, vol. 29, no. 3, pp. 297–302, Mar. 1999. https://doi.org/10.1016/S0008-8846(98)00207-5
M. Keppert, E. Vejmelková, P. Bezdička, M. Doleželová , M. Čáchová, L. Scheinherrová, J. Pokorný, M. Vyšvařil, P. Rovnaníková & R. Černý, “Red-clay ceramic powders as geopolymer precursors: Consideration of amorphous portion and CaO content,” Appl Clay Sci, vol. 161, pp. 82–89, Sep. 2018. https://doi.org/10.1016/j.clay.2018.04.019
M. Singh & M. Garg, “Making of anhydrite cement from waste gypsum,” Cem Concr Res, vol. 30, no. 4, pp. 571–577, Abr. 2000. https://doi.org/10.1016/S0008-8846(00)00209-X
F. Pacheco-Torgal, J. Castro-Gomes & S. Jalali, “Properties of tungsten mine waste geopolymeric binder,” Constr Build Mater, vol. 22, no. 6, pp. 1201–1211, Jun. 2008. https://doi.org/10.1016/j.conbuildmat.2007.01.022
P. Soares, A. T. Pinto, V. M. Ferreira & A. J. Labrincha, “Geopolímeros basados en residuos de la producción de áridos ligeros,” Mat Const, vol. 58, no. 291, pp. 23–34, Jul. 2008. Disponible en https://materconstrucc.revistas.csic.es/index.php/materconstrucc/article/view/105/144
A. Gharzouni, B. Samet, S. Baklouti, E. Joussein & S. Rossignol, “Addition of low reactive clay into metakaolin-based geopolymer formulation: Synthesis, existence domains and properties,” Powder Technol, vol. 288, pp. 212–220, Jan. 2016. https://doi.org/10.1016/j.powtec.2015.11.012
N. Hamdi, I.B. Messaoud & E. Srasra, “Production of geopolymer binders using clay minerals and industrial wastes,” C R Chim, vol. 22, no. 2–3, pp. 220–226, 2019. https://doi.org/10.1016/j.crci.2018.11.010
İ. Ustabaş & A. Kaya, “Comparing the pozzolanic activity properties of obsidian to those of fly ash and blast furnace slag,” Constr Build Mater, vol. 164, pp. 297–307, Mar. 2018. https://doi.org/10.1016/j.conbuildmat.2017.12.185
S. Top, H. Vapur, M. Altiner, D. Kaya & A. Ekicibil, “Properties of fly ash-based lightweight geopolymer concrete prepared using pumice and expanded perlite as aggregates,” J Mol Struct, vol. 1202, Feb. 2020. https://doi.org/10.1016/j.molstruc.2019.127236
N. Eroshkina & M. Korovkin, “The Effect of the Mixture Composition and Curing Conditions on the Properties of the Geopolymer Binder Based on Dust Crushing of the Granite,” Procedia Eng, vol. 150, pp. 1605–1609, Jan. 2016. https://doi.org/10.1016/j.proeng.2016.07.137
K.M.A. Hossain, “Properties of volcanic pumice based cement and lightweight concrete,” Cem Concr Res, vol. 34, no. 2, pp. 283–291, Feb. 2004. https://doi.org/10.1016/j.cemconres.2003.08.004
J. Davidovits, “Soft Minerallurgy and Geopolymers,” proceedings of the 1st International Conference on Geopolymer, ICG'88, Compiegne, FR, 1–3 Jun. 1988.
J. G. S. Van Jaarsveld, J. S. J. Van Deventer & L. Lorenzen, “Factors affecting the immobilization of metals in geopolymerized fly ash,” Metall Mater Trans B, vol. 29, no. 1, pp. 283–291, Feb. 1998. https://doi.org/10.1007/s11663-998-0032-z
T. Bakharev, “Durability of geopolymer materials in sodium and magnesium sulfate solutions,” Cem Concr Res, vol. 35, no. 6, pp. 1233–1246, Jun. 2005. https://doi.org/10.1016/j.cemconres.2004.09.002
D. L. Y. Kong, J. G. Sanjayan & K. Sagoe-Crentsil, “Comparative performance of geopolymers made with metakaolin and fly ash after exposure to elevated temperatures,” Cem Concr Res, vol. 37, no. 12, pp. 1583–1589, Dec. 2007. https://doi.org/10.1016/j.cemconres.2007.08.021
X. Guo, H. Shi & W.A. Dick, “Compressive strength and microstructural characteristics of class C fly ash geopolymer,” Cem Concr Compos, vol. 32, no. 2, pp. 142–147, Feb. 2010. https://doi.org/10.1016/j.cemconcomp.2009.11.003
M. Jin, F. Lian, R. Xia & Z. Wang, “Formulation and durability of a geopolymer based on metakaolin/tannery sludge,” Waste Manag, vol. 79, pp. 717–728, Sep. 2018. https://doi.org/10.1016/j.wasman.2018.08.039
M. Vafaei, A. Allahverdi, P. Dong & N. Bassim, “Acid attack on geopolymer cement mortar based on waste-glass powder and calcium aluminate cement at mild concentration,” Constr Build Mater, vol. 193, pp. 363–372, Dec. 2018. https://doi.org/10.1016/j.conbuildmat.2018.10.203
Método de ensayo para determinar la finura del cemento hidráulico por medio de los tamices 75 µm (M200) y 150 µm (M100), NTC 226, ICONTEC, 1998. Disponible en https://www.coursehero.com/file/41268695/NTC226PDF/
Gravedad específica y absorción de agregados finos, INV E-222, INVIAS, 2007. Disponible en https://es.slideshare.net/UCGcertificacionvial/gravedad-especfica-y-absorcin-de-agregado-fino
Análisis granulométrico de suelos por tamizado, INV E-123, INVIAS, 2007. Recuperado de http://www.lms.uni.edu.pe/labsuelos/MODOS%20OPERATIVOS/Analisis%20granulometrico%20por%20tamizado.pdf
Valor de azul de metileno en agregados finos y en llenante mineral, INV E-235, INVIAS, 2007. Disponible en https://es.scribd.com/document/111282589/INV-E-235-07-Azul-de-Metileno
Determinación de la densidad del cemento hidráulico, NTC 221, ICONTEC, 1998. Recuperado de https://tienda.icontec.org/wp-content/uploads/pdfs/NTC221.pdf
Método para determinar la resistencia a la compresión de morteros de cemento hidráulico usando cubos de 50 mm de lado, NTC 220, ICONTEC, 1998. Recuperado de https://kupdf.net/download/ntc-220-determinacion-de-la-resistencia-de-morteros-de-cemento-hidraulico-usando-cubos-de-50-mm-o-508-mm-de-lado_5b032c61e2b6f5475424a809_pdf
Instruments de pesaje de funcionamiento no automáticos. Requisitos metrológicos y Técnicos. Pruebas, NTC 2031, ICONTEC, 2014. Recuperado de https://members.wto.org/crnattachments/2016/TBT/COL/16_1549_01_s.pdf
R. Thenmozhi, R.V. Lakshmi, A.A. Francis & B.M. Meenal, “Strength behaviour of light weight aggregate based geopolymer concrete,” IJIRSET, vol. 7, no. 5, pp. 4755–4762, May. 2018. Available: http://www.ijirset.com/upload/2018/may/51_Strength.pdf
K. L. Aughenbaugh, T. Williamson & M. C. G. Juenger, “Critical evaluation of strength prediction methods for alkali-activated fly ash,” Mater Struct, vol. 48, no. 3, pp. 607–620, Mar. 2015. https://doi.org/10.1617/s11527-014-0496-z
M. C. Rowe, B.S. Ellis & A. Lindeberg, “Quantifying crystallization and devitrification of rhyolites by means of X-ray diffraction and electron microprobe analysis,” Am Min, vol. 97, no. 10, pp. 1685–1699, Oct. 2012. https://doi.org/10.2138/am.2012.4006
C. N. Achilles, R. V. Morris, S. J. Chipera, D. W. Ming and E. B. Rampe, “X-ray diffraction reference intensity ratios of amorphous and poorly crystalline phases: Implications for CheMin on the Mars Science Laboratory mission,” presented at 44th LPSC, LPSC, Wol, USA, 18–22 Mar. 2013. Available: https://www.lpi.usra.edu/meetings/lpsc2013/pdf/3072.pdf
O. Gencel, “Characteristics of fired clay bricks with pumice additive,” Energy Build, vol. 102, pp. 217–224, Sep. 2015. https://doi.org/10.1016/j.enbuild.2015.05.031
M. Malakootian, S. Bahraini, M. Malakootian & M. Zarrabi, “Removal of tetracycline antibiotic from aqueous solutions using natural and modified pumice with magnesium chloride,” Adv Environ Biol, vol. 10, no. 8, pp. 46–56, Sep. 2016. https://doi.org/10.17795/jjhr-37583
M. Heydari, K. Karimyan, M. Darvishmotevalli, A. Karami, Y. Vasseghian, N. Azizi, M. Ghayebzadeh & M. Moradi, “Data for efficiency comparison of raw pumice and manganese-modified pumice for removal phenol from aqueous environments - Application of response surface methodology,” Data Br, vol. 20, pp. 1942–1954, Oct. 2018. https://doi.org/10.1016/j.dib.2018.09.027
L. Gündüz, A. Sariisik, M. Davraz, D. Ugur & O. Cankiran, Pumice Technology, ISE, TR: SDU, 1998.
L. Gutiérrez, “El concreto y otros materiales para la construcción. MZL, CO: UNAL, 2003.
K. M. A. Hossain, “Properties of volcanic pumice based cement and lightweight concrete,” Cem Concr Res, vol. 34, no. 2, pp. 283–291, Feb. 2004. https://doi.org/10.1016/j.cemconres.2003.08.004
S. Yazicioğlu & B. Demğrel, “The Effect of the Pumice of Elazığ Region used as a Pozzolanic Additive on the Compressive Strength of Concrete in Increasing Cure Ages,” FUMBD, vol. 18, no. 3, pp. 367–374, Jan. 2006.
M. E. Valenzuela, “Estudio experimental de las propiedades de geoplímeros sintetizados a partir de puzolana natural,” memoria, dpto Ing Civil, UCh, SCL, CL, 2013. Disponible en http://repositorio.uchile.cl/handle/2250/114679
M. M. Yadollahi, A. Benli & R. Demirboğa, “Effects of elevated temperature on pumice based geopolymer composites,” Plast Rubber Compos, vol. 44, no. 6, pp. 226–237, Dec. 2015. https://doi.org/10.1179/1743289815Y.0000000020
E. Caballero, W. Sánchez & C.A. Ríos, “Síntesis de geopolímeros a partir de la activación alcalina de residuos de minería del oro,” Ing Compet, vol. 16, no. 1, pp. 317–330, Jan. 2014. hhttps://doi.org/10.25100/iyc.v16i1.3735
N. Değirmenci & A. Yilmaz, “Use of pumice fine aggregate as an alternative to standard sand in production of lightweight cement mortar,” IJEMS, vol. 18, pp. 61–68, Feb. 2011. Available: http://nopr.niscair.res.in/bitstream/123456789/11207/1/IJEMS%2018(1)%2061-68.pdf
K. M. A. Hossain, “Pumice based blended cement concretes exposed to marine environment: Effects of mix composition and curing conditions,” Cem Concr Compos, vol. 30, no. 2, pp. 97–105, Feb. 2008. https://doi.org/10.1016/j.cemconcomp.2007.05.013
H. Binici & O. Aksogan, “Sulfate resistance of plain and blended cement,” Cem Concr Compos, vol. 28, no. 1, pp. 39–46, Jan. 2006. https://doi.org/10.1016/j.cemconcomp.2005.08.002
I. B. Messaoud, N. Hamdi & E. Srasra, “Physicochemical Characterization of Geopolymer Binders and Foams Made from Tunisian Clay,” Adv Mater Sci Eng, vol. 2018, pp. 1–8, Feb. 2018. https://doi.org/10.1155/2018/9392743
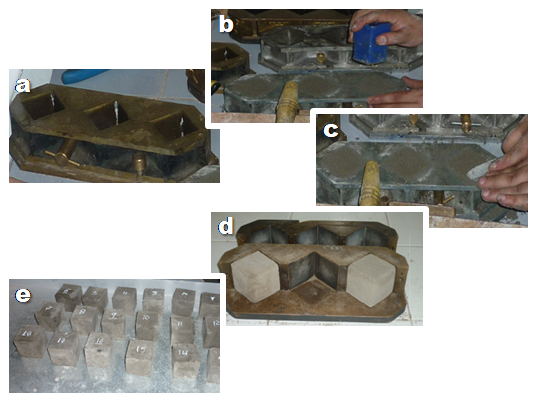
Publicado
Cómo citar
Número
Sección
Licencia
Derechos de autor 2021 INGE CUC

Esta obra está bajo una licencia internacional Creative Commons Atribución-NoComercial-SinDerivadas 4.0.
Los artículos publicados son de exclusiva responsabilidad de sus autores y no reflejan necesariamente las opiniones del comité editorial.
La Revista INGE CUC respeta los derechos morales de sus autores, los cuales ceden al comité editorial los derechos patrimoniales del material publicado. A su vez, los autores informan que el presente trabajo es inédito y no ha sido publicado anteriormente.
Todos los artículos están bajo una Licencia Creative Commons Atribución-NoComercial-SinDerivadas 4.0 Internacional.